Solar plasmas
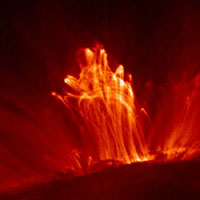
Tangled loops in the solar corona.
Credit: TRACE/NASA; courtesy of LMSAL.
"Plasma", consisting of a sea of positively-charged ions and negatively-charged electrons, is sometimes called the fourth state of matter, and more than 99% of the matter in the universe is in the plasma state. The Solar Plasma Group researches into plasma processes in the Sun and beyond, developing mathematical models of the complex interactions between plasmas and magnetic fields.
Some key research questions are:
- How is plasma in the solar corona heated to temperatures of millions of degrees?
- How does the process of magnetic reconnection work in the solar corona and elsewhere?
- What is the origin of high energy charged particles in solar flares?
We are also involved in modelling the interaction of plasma with magnetic fields in magnetically-confined fusion devices such as tokamaks and spheromaks.
Details of research projects
Our research is supported by grants from STFC and also by UKAEA Fusion. We have active collaborations with groups in the UK and internationally, including Belgium, France, Japan, Russia and USA.
We are mainly concerned with modelling the nature and effects of magnetic reconnection in the solar atmosphere, with particular relevance to coronal heating and solar flares. Magnetic reconnection is a process of fundamental importance in astrophysics, with relevance ranging from galactic magnetic fields, to stellar and solar activity, to planetary magnetospheres. It also is important in magnetically-confined fusion plasmas. In the case of the Sun, its role has become even more evident after spectacular observations of the solar corona with the Yohkoh/SXT telescope as well as with more recent SoHO, TRACE and Hinode satellites. In particular, magnetic reconnection is crucial to both flares and coronal heating. Both MHD and kinetic plasma approaches are used. This theoretical research underpins developments from recent space borne solar observatories such as SoHO, TRACE, Hinode, RHESSI and Stereo. We are also involved in studies of magnetically-confined fusion plasmas.
Solar flares are dramatic and complex events which are of interest both in their own right and because of their significant effects on the Earth's space environment by shaping what is now called "space weather". A solar flare involves the release of up to 1025 Joules of stored magnetic energy, in timescales of minutes-hours, with signatures right across the electromagnetic spectrum. There is now a considerable body of evidence demonstrating that magnetic reconnection is the fundamental process of energy release in solar flares. A key aspect of flares is the generation of large numbers of high energy charged particles, and we are developing models to explain their origin.
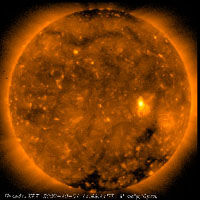
The solar corona in X-rays.
Credit: Hinode/XRT.
A major focus of our current research is the solar coronal heating problem, a major unsolved problem of astrophysics. How is coronal plasma maintained at temperatures of millions of degrees Kelvin, while the surface temperature is only a few thousand degrees? It is now accepted that the coronal magnetic field is integral to the heating process, but the details remain controversial. A promising candidate for effective dissipation of energy is magnetic reconnection, the same process which is responsible for the energy release in solar flares. Whilst these large events are too infrequent to heat the bulk corona, it has been proposed that coronal heating is due to the combined effect of many smaller events, known as nanoflares. One aim of our work is to predict the properties and occurrence rate of such nanoflares, and to determine the observational consequences of nanoflare heating.
Forced magnetic reconnection in the solar corona
Forced reconnection occurs when an external disturbance at the boundary of a plasma triggers the formation of a current sheet with subsequent energy release by magnetic reconnection. We have developed linear analytical models of forced reconnection both for a single pulse and oscillatory driving, as well as numerical MHD simulations of nonlinear aspects. These confirm the major theoretical conclusion that the role of an external perturbation is just to trigger the process, while the released energy is tapped from the energy excess accumulated in the initial magnetic configuration. Therefore, even a weak and energetically minor external influence can result in a major magnetic relaxation of a system, which may have important implications for understanding magnetic explosive events such as solar flares
Ongoing research is focussing on improving understanding of the role of the two-fluid effects such a the Hall effect in forced and spontaneous (tearing instability) magnetic reconnection.
Particle acceleration via magnetic reconnection
There are several forms to which excess magnetic energy released via reconnection can be converted: plasma heating, kinetic energy of the plasma flow , energisation of high energy particles. The latter is due to an inductive electric field associated with reconnection. So long as the population of energetic particles is small, the test particle approach can be used. We have utilised this approach to study particle acceleration at reconnecting 3D null points, which should be quite common in the solar corona. We are also investigating particle acceleration during forced reconnection, enabling us to follow particle trajectories globally, and to link this process to the external driving.
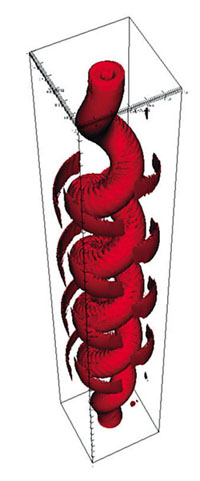
A computer simulation of a coronal loop with a nonlinear kink instability.
Credit: Philippa Browning, JBCA.
Magnetic relaxation, kink instabilities and nanoflares
We have developed a model which predicts the onset and energy release in nanoflares. Thus, a nanoflare, or similar event, occurs when the coronal magnetic field becomes unstable to ideal current-driven instabilities such as kink instability, while energy is released by magnetic reconnection as current sheets form in the nonlinear phase of the instability. The energy release may be very effectively estimated by assuming a helicity-conserving relaxation to a minimum energy state. We have shown that this leads to heating events with a wide range of sizes. We are also investigating the details of the nonlinear kink instability and the relaxation process by using 3D numerical simulations.
Detection of nanoflares
As is well known from the hard X-ray observations, the occurrence rate of flares as a function of their energy obeys an inverse power-law relationship with a power-law index α ≈ 1.5-1.7 that remains unchanged over a wide energy range of 1028 - 1031 erg, while for nanoflares to be energetically significant in the context coronal heating, these smaller events must have a steeper energy distribution with an index α > 2. Efforts to determine α from analysis of individual events have proved inconclusive, which motivated us to develop another approach to this problem. The methodology is based on the statistical interpretation of the variability in the coronal EUV and soft X-ray emission. Indeed, discreteness of heating events (nanoflares) should inevitably result in fluctuations in the coronal heating power and, hence, in the intensity of its radiation observed as transient brightening. The observational data were compared with a Monte-Carlo type numerical simulation code, which made it possible to estimate the energy and occurrence rate of nanoflares in an Active Region observed with Yohkoh/SXT. However, further dedicated studies of available observations and their comparison with theoretical predictions are necessary to obtain reliable results on nanoflare properties. Therefore, we are extending the methodology to a wider set of X-ray and EUV data, in particular to those obtained with TRACE and Hinode, that have much better temporal and spatial resolution, making use of the complementary properties of the different instruments.
Magnetically-confined fusion plasmas
We have a long established programme of research into magnetically-confined fusion plasmas; the physical processes involved have strong similarities with astrophysical plasmas. One project is concerned with the interactions of plasma with neutral gas in a linear divertor simulator device, with relevance to tokamak divertors. In particular, we are concerned with the role of different modes of recombination in detachment. We are also studying Edge Localised Modes (ELMs) in tokamaks, developing a model based on relaxation theory to predict ELM sizes. Finally, we are investigating the role of instabilities in helicity-injection current drive in spheromaks and spherical tokamaks. The underlying idea is that current-driven instabilities on open flux regions, which are directly connected to the driving electrodes, lead to fluctuations that affect the relaxation process and the transfer of helicity onto the closed flux domain.